Discovering the Higgs: Inevitability, Rigidity, Fragility, Beauty
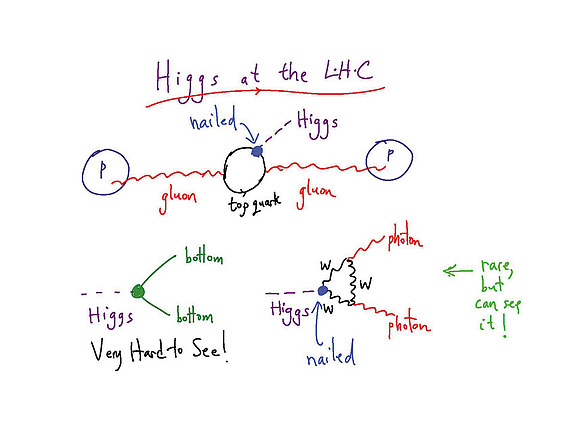
Following the discovery in July of a Higgs-like boson—an effort that took more than fifty years of experimental work and more than 10,000 scientists and engineers working on the Large Hadron Collider—Juan Maldacena and Nima Arkani-Hamed, two Professors in the School of Natural Sciences, gave separate public lectures on the symmetry and simplicity of the laws of physics, and why the discovery of the Higgs was inevitable.
Peter Higgs, who predicted the existence of the particle, gave one of his first seminars on the topic at the Institute in 1966, at the invitation of Freeman Dyson. “The discovery attests to the enormous importance of fundamental, deep ideas, the substantial length of time these ideas can take to come to fruition, and the enormous impact they have on the world,” said Robbert Dijkgraaf, Director and Leon Levy Professor.
In their lectures “The Symmetry and Simplicity of the Laws of Nature and the Higgs Boson” and “The Inevitability of Physical Laws: Why the Higgs Has to Exist,” Maldacena and Arkani-Hamed described the theoretical ideas that were developed in the 1960s and 70s, leading to our current understanding of the Standard Model of particle physics and the recent discovery of the Higgs-like boson. Arkani-Hamed framed the hunt for the Higgs as a detective story with an inevitable ending. Maldacena compared our understanding of nature to the fairytale Beauty and the Beast.
“What we know already is incredibly rigid. The laws are very rigid within the structure we have, and they are very fragile to monkeying with the structure,” said Arkani-Hamed. “Often in physics and mathematics, people will talk about beauty. Things that are beautiful, ideas that are beautiful, theoretical structures that are beautiful, have this feeling of inevitability, and this flip side of rigidity and fragility about them.”
The recent discovery of the Higgs-like boson is “a triumph for experiment but also a triumph for theory,” said Arkani-Hamed. “We were led to saying, ‘This thing has got to be there. We’ve never seen one before, but by these arguments, by our little detective story, it’s gotta be there.’ And by God, it is. It’s allowed to be there. It can be there. It is there.”
In Maldacena’s comparison, beauty is the fundamental forces of nature—gravity, electromagnetism, the strong force, and the weak force—and the beast is the Higgs mechanism. “We really need both to understand nature,” said Maldacena. “We are, in some sense, the children of this marriage.”
Current knowledge of the fundamental forces of physics is based on two well established theories: the Standard Model of particle physics, a set of equations that gives an impressively accurate description of elementary particles and their interactions, but omits gravity and only accounts for about one-sixth of the matter in the universe; and Einstein’s theory of general relativity, which describes the observed gravitational behavior of large objects in the universe, such as galaxies and clusters of galaxies, but has yet to be reconciled with quantum principles.
Ordinary matter—the material we see and are familiar with, such as the planets, the stars, human bodies, and everyday objects—is acted on by gravity, electromagnetism, the strong force, and the weak force. These interactions apply over an enormous range of distances—from the size of the observable universe (around 1028 centimeters) down to the weak scale (around 10–17 centimeters).
In the Standard Model of particle physics, nature is built out of elementary building blocks, such as electrons and quarks. Forces between particles are transmitted by other particles, such as photons, the carrier of electromagnetic forces, and W and Z particles, the basic particles that transmit the weak interactions. The Higgs isn’t the first particle that the Standard Model has predicted and that has been later discovered experimentally. The model also has led to the prediction and discovery of the W and Z particles, the top quark, and the tau neutrino.
The Higgs boson explains how most fundamental particles acquire mass as they interact with a Higgs field that exists everywhere in the universe. It is the final element of the Standard Model that needed to be confirmed experimentally and its discovery promises to provide further understanding of the origin of mass and help clarify some long-standing mysteries.
The weak scale is the distance that is being probed at the Large Hadron Collider, where the Higgs-like boson was discovered. With all ordinary matter and interactions, the force between two electrons (the size of the quantum mechanical fluctuations) gets weaker as you go to longer distances (lower energies) and stronger at shorter distances (higher energies), a basic consequence of the Heisenberg uncertainty principle.
“We’ve learned that the essential unity and simplicity of the laws of nature become manifest at short distances,” explained Arkani-Hamed. “They’re hidden at large distances by a variety of accidents, but when we go to short distances we finally see them. We see for the first time all these different interactions described in a common way.”
In the Standard Model, all particles intrinsically have some spin and an angular momentum that is associated with that spin. Known particles have angular momenta, measured in H-bar (Planck’s constant) units, in multiples of 1/2. According to the model, the only allowable spins are 0, 1/2, 1, 3/2, and 2, but we have seen only a subset of that: 1/2, 1, and 2. The electron has spin 1/2. The photon has spin 1. The graviton, which interacts the same with everything, is the only particle that has spin 2.
The story of the Higgs starts by trying to understand why some particles have mass. According to the Standard Model, the W and Z particles that carry the electroweak force should have zero mass to allow for the unification of the electromagnetic and weak nuclear forces in a single electroweak force. Between theory and experiment, it was determined that the Higgs particle had to enter the picture under 200 GeV (a unit to measure mass), that it had to interact with W, Z, and top quark particles, and that it had to have 0 spin. While the Standard Model did not predict the exact mass of a Higgs particle, from precise measurements, it was known that it had to be somewhere between 80 to around 200 times the mass of a proton. The Higgs-like boson, which was discovered last summer in the mass region of around 126 GeV, allows once-massless particles to have mass without destroying the principles of the Standard Model.
“People sometimes ask, what is this [the discovery of the Higgs] useful for?” said Maldacena. “I have to be honest, I don’t know of any technological application. There is the apocryphal quote of [Michael] Faraday. When asked what the possible technological application of electricity was, he said to the prime minister, ‘Someday we will be able to tax it.’ I think, maybe, we could say the same thing about the Higgs boson. Something we do know is that it is helping us understand what happens in nature at very short distances.”
Gauge symmetries determine the interactions and production of particles, and Maldacena used a monetary analogy to describe the gauge symmetries of the electromagnetic and weak force. In his analogy, the magnetic field is a gauge symmetry where each country is identical except they can choose their own currency. All money must be changed to the new currency when moving from country to country.
In physics, the currency is the rotations within a circle at each point in spacetime, and the exchange rate is the electromagnetic potential, or the displacement that results from traveling from one small spacetime region (country) to the next. Following a quantum mechanic understanding of the probabilistic laws of nature, “these exchange rates are random with a probabilistic distribution that depends on the opportunity to speculate,” said Maldacena. “Nature doesn’t like speculation, and will not offer you these opportunities very easily, but it will offer them to you, if you can find them.”
The gauge symmetry of weak interactions involves symmetries of spheres rather than circles at each point in spacetime. Maldacena described the Higgs mechanism as an object sitting at each point on these weak spheres. When a rotation is made—even in a vacuum and empty space—this mechanism causes a transformation or change.
Continuing the monetary analogy, Maldacena introduced the notion of being able to buy something, in this case gold, in each country. The gold can be taken from one country to the next, its price is set by each of the countries, and money can be earned by going back and forth between the countries. In this analogy, the price of gold in each country is the Higgs field. Once the price or gauge is set to a constant value everywhere in space, this leads to a preferential value for the exchange rates, and leads to the masses for the W and Z weak bosons. In Maldacena’s analogy, the Higgs boson arises when there are two objects, such as gold and silver, to purchase. The relative price of gold and silver is the Higgs boson; the ratio behaves as a massive particle. According to Maldacena, it is necessary to have at least two objects to buy so that when the distances between points in spacetime becomes very small we can still retain interesting interactions at long distances.
The Higgs-like boson was produced at the LHC in an indirect way but according to similar gauge symmetries derived from the Standard Model. When protons collide, they produce many particles. Very rarely, they produce Higgs bosons. These Higgs bosons decay very quickly into particles, such as two photons. Since the Higgs bosons decay too quickly to discern, theorists predicted that experimentalists could detect the Higgs by looking at events that have two photons and finding a bump in the data where two photons would amount to the mass of the Higgs boson.
The Higgs boson is the first particle with spin 0. This leaves only spin 3/2 unrealized in nature. But there is a strong candidate. Supersymmetry is associated with 3/2, and it is possible that the LHC will confirm the existence of supersymmetry, which extends the Standard Model and unites matter particles and force particles by pairing them in a single framework. It suggests that the strong force, the weak force, and the electromagnetic force become one at very short distances.
Supersymmetry also naturally leads to a new dark matter particle that does not emit or absorb light, and can only be detected from its gravitational effects. Ordinary matter that is explained by the Standard Model makes up about 4 percent of the universe; dark matter comprises about 22 percent.
“We know from astrophysical observations that there is more matter than what we see,” said Maldacena.
“If we look at the sky, we see some galaxies sitting there in the sky, surrounded by what looks like the blackness of empty space. What we don’t know is whether this dark matter particle will or will not be produced at the LHC.“
In the last decade, astronomical observations of several kinds, particularly of distant supernova and the cosmic microwave background, also indicate the existence of what is known as dark energy, a uniform background field that makes up about 74 percent of the universe and is credited with accelerating the expansion of the universe. The presence of dark energy suggests a fundamental gap in our current understanding of the basic forces of nature.
“Space, time, and quantum mechanics framed the central dramas of the twentieth century, and really have taken us shockingly far. The story of the Higgs is the last example of how far they took us. But in a sense, the story of the Higgs is one of the last embers of the set of ideas that we dealt with and understood in the twentieth century,” said Arkani-Hamed.
“Relativity and quantum mechanics—the picture of spacetime that Einstein gave us and quantum mechanics—are incredibly rigid and powerful. The next set of questions is: Where do these things come from? That’s the one thing I didn’t question. I just took spacetime and quantum mechanics and the rest of it followed. What is the deeper origin of spacetime and quantum mechanics? This is what you should ask your friendly neighborhood string theorist.”
Recommended Viewing: A video of Juan Maldacena's lecture, "The Symmetry and Simplicity of the Laws of Nature and the Higgs Boson," is available at https://www.ias.edu/video/maldacena-lecture-10-12; a video of Nima Arkani-Hamed's lecture, "The Inevitability of Physical Laws: Why the Higgs Has to Exist," is available at https://www.ias.edu/video/arkani-hamed-lecture-10-12.