Nima Arkani-Hamed: Unraveling Nature’s Mysteries
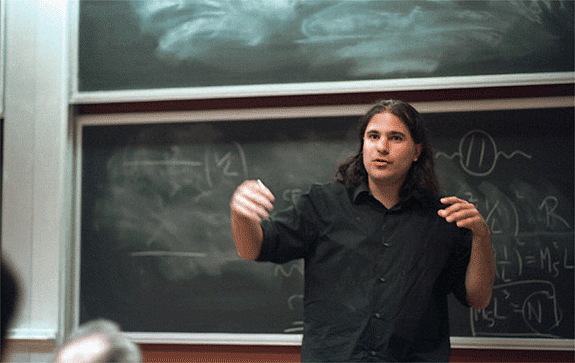
“Everything here is fraught with danger and excitement,” says Nima Arkani-Hamed, Professor in the School of Natural Sciences. With a broad sweep of his hand, he motions to the diagram he has drawn on the chalkboard in his office of the range of distance scales for known phenomena—from 10–33 cm, which is associated with quantum gravity and string theory, to 10+28 cm, which is the size of the universe.
“Why is the universe big, why is gravity so weak? You would think after 2,000 years of thinking about physics we would have good answers to questions like that. We have lousy answers to these questions,” says Arkani-Hamed. “Our current laws of nature—the Standard Model of particle physics—are perfectly consistent. No experiments contradict them, but they give such lousy answers to these questions that we think we are missing something very, very big.”
With the imminent start-up of the Large Hadron Collider (LHC), a particle accelerator that will collide protons together and allow us to probe the laws of nature down to distances of 10–17 cm, a billion times smaller than the atom, and ten times smaller than the tiniest distances we have probed to date, fundamental particle physics is on the threshold of a new era.
Arkani-Hamed, one of the world’s leading phenomenologists who joined the Faculty in January, has taken a lead in building models of the universe that relate to theories that can be tested at the LHC—from supersymmetry to large extra dimensions of space to the idea that our universe exists in a sea of universes, each governed by a different set of principles.
“I try to take ideas that are in the theoretical zeitgeist and see if they might be relevant to solving any of the outstanding mysteries, and then see what experimental consequences can be derived,” says Arkani-Hamed. “Phenomenologists are jacks of all trades. We try to propose theories that extend things that we have seen, figure out the direct observational consequences of those theories, and work closely with our experimental colleagues to see how we can actually extract information about nature directly from an experiment.”
Among the ideas that will be tested at the LHC is the existence of supersymmetry, which involves the ordinary dimensions of space and time having quantum mechanical partners, and the possibility that there may be extra spatial dimensions aside from the three spatial dimensions familiar to us. Both supersymmetry and extra dimensions are essential components of string theory, the leading candidate for unifying general relativity and quantum mechanics. These are all subjects that Institute physicists have taken a lead in developing.
Just as for every particle there exists an antiparticle, supersymmetry predicts that for every known particle there also exists a superpartner particle. Part of the strong theoretical appeal of supersymmetry is its possible connection to dark energy and the fact that it provides a natural candidate for dark matter—a new weakly interacting massive particle (WIMP) with mass close to the scale that will be probed at the LHC.
“Often people will describe the LHC or accelerators in general as microscopes for probing short distances. But normally, a microscope is looking at something. What is the LHC looking at? It is looking at the vacuum,” says Arkani-Hamed. “People like to say the dark energy is very mysterious and we don’t know what it is but that is a bit of an exaggeration, because there is an extremely simple thing that it could be. It could be the energy of the vacuum. It’s not that we don’t know how to accommodate it in our equations. We definitely know how to accommodate it. The problem is that we get it 120 orders of magnitude bigger than it apparently is.”
To accommodate dark energy in particle physics requires unnatural fine-tuning, which also arises in another aspect of Arkani-Hamed’s research—a paradox of the Standard Model called the “hierarchy problem” that relates to the extreme weakness of gravity in comparison to the other forces of nature— electromagnetism, the strong nuclear force, and the weak nuclear force. Violent short distance quantum fluctuations in the vacuum would naturally lead to the prediction that the strength of gravity is thirty orders of magnitude larger than its observed strength, requiring inordinate fine-tuning for the parameters of the theory.
“Fine-tuning is like walking into a room and seeing a pencil standing on its tip in the middle of a table,” says Arkani-Hamed. “If you saw it, you would think that maybe there is a little string hanging from the ceiling that you missed, or maybe there is a little hand holding it up or something. The dark energy problem and the hierarchy problem are conceptually identical puzzles. In both cases, we have to do a tremendous amount of fine-tuning in order to explain some very obvious property of our world because we don’t yet see any dynamics or any mechanism for explaining why it is what it is.”
Particle physics data point to another mysterious component of empty space, the Higgs field, a force that fills space and gives particles the property of mass and might be related to dark energy. Arkani-Hamed is willing to bet several months’ salary that the Higgs particle, the last element predicted by the Standard Model that has not been confirmed experimentally, will be discovered at the LHC.
While supersymmetry is the most popular solution to the hierarchy problem, Arkani-Hamed has proposed other possibilities, including the existence of large extra dimensions of space, which dilute gravity’s strength, and a theory called split supersymmetry, in which only half of all particles have superpartners. “One of the confusing things about supersymmetry,” says Arkani-Hamed, “is that people have mounted tons of experiments to look for possible signals of these partner particles and so far there has been no hint of it directly or indirectly.”
Split supersymmetry finds a common explanation for the cosmological constant and the hierarchy problem. It relates to the theory of the multiverse, in which our entire observable universe might be a tiny part of a much larger multiverse, in which many universes function according to distinct and self-containing physical laws with one common exception: gravity, which can travel freely between them. “This is a very controversial idea, because to invoke universes you can’t see to explain properties of our own universe is obviously a tricky proposition,” says Arkani-Hamed. “But it is not obviously wrong. It is a subject of lots of continuing activity and thinking right now.”
In a multiverse, a near-infinite number of universes exist, but ours is the only one we can observe because it is the only one in which we can live—a concept also known as the anthropic principle. “It is very interesting that the observed value of the cosmological constant, the observed value of the vacuum of dark energy, if you interpret the dark energy as a cosmological constant, is right around the value where if it was a little bigger then the universe would be empty,” says Arkani-Hamed.
In his recent talk on dark energy at the Space Telescope Science Institute, Edward Witten, Charles Simonyi Professor in the School of Natural Sciences, addressed the theoretical possibility of a multiverse in which the aim is not to explain why the vacuum has a very tiny energy but rather to look for a theory that generates all kinds of vacua with different properties that are realized in different times and places in a multiverse, perhaps as a result of cosmic inflation.
The good news, if we are living in a multiverse in which the only vacuum we can observe is the one that allows our existence, Witten says, is that the Standard Model as we know it may be fairly accurate. “If the universe is really a multiverse, finding the vacuum state we observe should be like searching for a needle in a haystack,” says Witten. “But this comes with a hefty dose of bad news: if the vacuum of the real world is really a needle in a haystack, it is hard to see how we are supposed to be able to understand it.”
At the Institute, Arkani-Hamed will be looking at LHC data to interpret signals that underscore these and other theoretical possibilities while helping to attract and mentor highly talented postdoctoral fellows, with a diversity of theoretical skills, in anticipation of a golden era of discovery. Speaking of his decision to come to the Institute from his position as Professor of Physics at Harvard, Arkani-Hamed says, “This is a very, very special group. I couldn’t possibly ask for better or more stimulating colleagues in high energy theory and string theory, quantum field theory, and astronomy.”
“Here I have the ability to really focus very sharply on doing science. Again, I keep bringing this up because it looms so large in most of our minds: an experiment like the LHC is a once-in-a-lifetime opportunity. There really seem to be very fundamental scientific issues at stake. And there really is a chance to unravel them and learn something potentially revolutionary and new about nature. You can’t give up on opportunities like that.”