Interstellar Magnetism
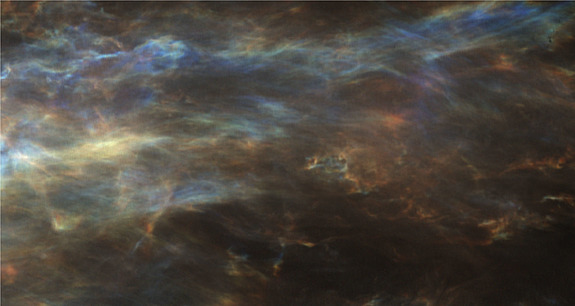
You may have done this experiment as a child: spread a bunch of iron filings on a table, a heap of insouciant metal dust. Now place a bar magnet in their midst, and ah! The iron filings snap to attention, as if endowed with a sudden sense of purpose. They align their lengths with the local magnetic field orientation, and suddenly an invisible presence is revealed: the curving contours of the magnetic field.
I want to pull off a similar revealing act. I want to see, and understand, the magnetic field that permeates our Milky Way galaxy.
From our perch here on Earth, we primarily learn about the magnetic field through its influence on light. One of the techniques we use is somewhat analogous to the iron filings experiment. Interstellar space is vast, tenuous, and dusty. Tiny dust grains float in the great expanses between stars. These dust grains are in general aspherical, and, torqued hither and yon by ambient radiation, they find a preferred orientation: they align their short axes with the local magnetic field.1,2 As starlight filters through interstellar dust, the aligned dust grains absorb a particular polarization of light, such that the starlight that finally arrives at our telescopes is linearly polarized in a direction determined by the orientation of the intervening magnetic field. The dust grains themselves radiate thermal emission at infrared wavelengths that is likewise polarized. So by observing starlight polarization and polarized dust emission, we measure a component of the interstellar magnetic field.
How else does interstellar magnetism reveal itself? My collaborators and I discovered a new way to trace the orientation of the magnetic field, through its influence on the structure of interstellar gas. Like dust, neutral hydrogen gas is pervasive in the interstellar medium—the stuff between the stars. With the unparalleled sensitivity of the Arecibo radio telescope in Puerto Rico, we revealed an imprint of the interstellar magnetic field on the texture of the diffuse interstellar medium.
Long, thin tendrils of neutral hydrogen stretch for parsecs across interstellar space. My colleagues and I showed that these “fibers” of neutral hydrogen are well aligned with the local magnetic field. The galactic magnetic field creates order in the turbulent space between the stars, and we can use the morphology of neutral gas to trace the magnetic field orientation. Importantly, these structures are seen projected onto the plane of the sky; stuck in our little corner of the galaxy, we view the Milky Way from a single vantage point. Neutral hydrogen orientation, like dust polarization and starlight polarization, can only tell us about a single component of the three-dimensional magnetic field we seek to map. However, in my first year at the Institute, I found a way to probe the line-of-sight component of the magnetic field using neutral hydrogen. I showed that measuring the orientation of the gas structures at different Doppler-shifted velocities probes how tangled the magnetic field is along the line of sight.3 Line-of-sight magnetic field tangling—differently oriented magnetic fields at different distances in a given direction—gives rise to phenomena like depolarization of the dust emission. This new probe of the coherence of the magnetic field lets us better understand how the field, and the gas and dust it permeates, are distributed in the galaxy.
We have also recently made progress in understanding what these magnetically aligned neutral hydrogen structures are. After our initial discovery, some researchers proposed that the observed magnetic alignment was a trick of the velocity field: that these were not real, three-dimensional neutral hydrogen structures in space, but simply a coincidence of turbulent velocity fields shifting emission to different frequencies. My collaborators and I recently published a paper showing that in fact these structures are “real”: dusty, three-dimensional features that seem to be colder on average than their surroundings.4 Much remains to be explored about how and why these cold neutral hydrogen structures are so anisotropic, and so deeply coupled to the magnetic field.
Understanding galactic magnetism is an exciting goal unto itself, but there lurks another motivation for this work, one that takes us far beyond our home galaxy. The question: what happened during the first trillionth of a trillionth of a billionth of a second after the Big Bang? It is currently thought that in this fraction of a second after popping into existence, the universe underwent a growth spurt: a period of extremely rapid expansion called inflation. While a number of observations are consistent with inflation, we have yet to detect a “smoking gun”: a signal uniquely predicted by an inflationary cosmology. The search is on for just such a signal: a pattern of polarization imprinted in the cosmic microwave background (CMB), the residual radiation from the formation of the universe. Measuring this signal, inflationary gravitational wave B-mode polarization, is the chief goal of many current and proposed experiments.
The principal difficulty in finding this polarization signal in the CMB is that we have to look through our galaxy. The tiny dust grains that let us probe the galactic magnetic field emit polarized radiation at the same frequencies where we observe the polarized CMB. The primordial signal is buried under a galactic signal that is far stronger. Or, as the saying goes, one person’s treasure is another person’s contaminating foreground. Our hope in solving the puzzle of inflation thus hinges on our ability to measure the polarized dust signal as precisely as possible, in order to separate it from the polarized CMB. To this end, I am now collaborating with Brandon Hensley, a postdoc at Princeton University and an expert in the microphysics of interstellar dust. We are using all of the insights gleaned over the past several years about how neutral hydrogen traces the magnetic field to build new, data-driven models of the polarized dust signal. Our model uses neutral hydrogen to probe both the plane-of-sky magnetic field and the variable magnetic field along the line of sight. It’s a new way of linking the magnetized gas in the interstellar medium to the dust foreground that obscures our view of the polarized CMB. We’re in pursuit of the invisible: mapping the elusive magnetic field between the stars. And the better we understand the magnetized interstellar medium, the better we will be able to peer back to the beginning of time.
1. “Magnetically Aligned HI fibers and the Rolling Hough Transform.” S. E. Clark, J. E. G. Peek, M. E. Putman. 2014, The Astrophysical Journal, 789, 82.
2. “Neutral Hydrogen Structures Trace Dust Polarization Angle: Implications for Cosmic Microwave Background Foregrounds.” S. E. Clark, J. C. Hill, J. E. G. Peek, M. E. Putman, B. L. Babler. 2015, Physical Review Letters, 115, 241302.
3. “A New Probe of Line-of-Sight Magnetic Field Tangling.” S. E. Clark. 2018, The Astrophysical Journal Letters, 857, L10.
4. “The Physical Nature of Neutral Hydrogen Intensity Structure.” S. E. Clark, J. E. G. Peek, M.-A. Miville-Deschênes. 2019, The Astrophysical Journal, 874, 171.