Life on Other Planets
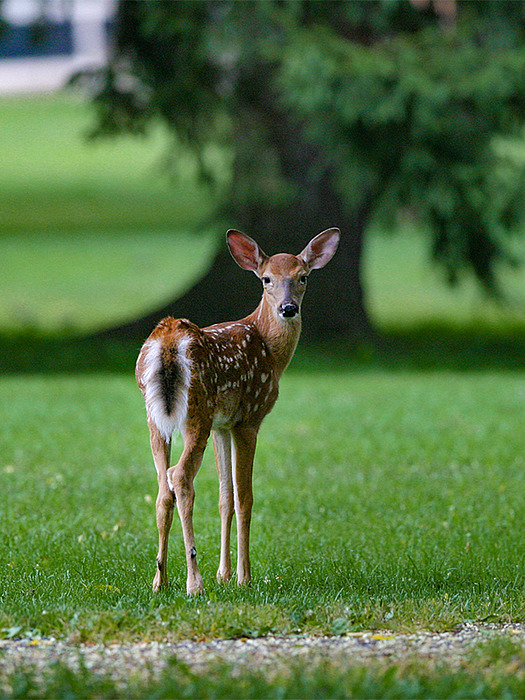
Until a couple of decades ago, the only planets we knew existed were the nine in our Solar System. In the last twenty-five years, we’ve lost one of the local ones (Pluto, now classified as a “minor planet”) and gained about three thousand candidate planets around other stars, dubbed exoplanets. The new field of exoplanetary science is perhaps the fastest growing subfield of astrophysics, and will remain a core discipline for the forseeable future.
The fact that any biology beyond Earth seems likely to live on such a planet is among the many reasons why the study of exoplanets is so compelling. In short, planets are not merely astrophysical objects but also (at least some of them) potential abodes.
The highly successful Kepler mission involves a satellite with a sensitive telescope/camera that stares at a patch of sky in the direction of the constellation Cygnus. The goal of the mission is to find what fraction of Sun-like stars have Earth-sized planets with a similar Earth-Sun separation (about 150 million kilometers, or the distance light travels in eight minutes).
Within the next several decades, with rapid progress in biology, with new space missions, and with large and sensitive new telescopes that are planned, it is conceivable that we might find any of these three kinds of independent life. In this way, we will be able to make a much more informed estimate of the frequency of life in the universe.
During its half-decade mission lifetime, Kepler will be monitoring 150,000 stars, looking for slight periodic dips in starlight that occur if an exoplanet’s orbital plane is oriented precisely along our line of sight. In this geometrical configuration, the planet moves directly between us and its parent star once per orbit, blocking a tiny fraction of the light from the star. Kepler has identified more than two thousand planet candidates so far, most of which are probably real. Early results suggest that somewhere between 5 percent and 50 percent of Sun-like stars probably have an approximate Earth-analog!
So, we are starting to realize that potential homes for life are probably common in our galaxy. Among the several hundred billion stars, there might be tens of billions of rocky planets located in the “habitable zones” of their stars—the regions where they would have roughly Earth-like temperatures. With so many possible places where life might flourish, how much life can we expect is out there? This question might seem to invite nothing but wild speculation. However, there is a potential avenue for making an estimate.
As an analogy, consider someone who wants to know what fraction of the time there is a deer visible outside her window. One way to estimate this would be to sit by the window, looking out, and see how long she has to wait for the first deer to walk into sight. In Manhattan, the expected wait might be decades, and one could rightly infer that the fraction of the time that there is a deer in sight is very close to zero. In Fuld Hall at IAS, one probably wouldn’t have to wait more than a few hours, and could rightly infer that deer are pretty frequently visible outside the window.
Similarly, we can look through the Earth’s geological history to see when life appeared in Earth’s history. How long, in other words, did Earth have to wait before life “walked into sight”? Earth was born about 4.5 billion years ago, but for the first half billion years of its existence, it was bombarded by impactors that probably sterilized it. For the past four billion years, though, the Earth has been essentially continuously habitable (meaning, it has had conditions suitable for liquid-water-based life). There is some evidence that the earliest living organisms had developed by 3.8 billion years ago, or within the first two hundred million years of the habitable history of the Earth. A common line of reasoning in the origin of life community argues that since abiogenesis (the process of life arising from abiotic conditions) occurred so early in the geological time scale of the Earth, it must be a reasonably probable process.
This argument is appealing, and it’s certainly true that the early emergence of life on Earth provides some reason for optimism for an enthusiast of extrasolar life. However, together with Professor Edwin Turner of Princeton University, I recently critically reevaluated this argument (in the Proceedings of the National Academy of Sciences, vol. 109, issue 2) and found that we have less reason to expect that the Galaxy is full of life than is sometimes assumed. One important reason is that there is a powerful selection effect in operation that is absent in the deer analogy. Specifically, what we know is more than simply that life showed up early; we also know that we are aware that life showed up early. Put differently, in order for us to exist, enough time had to have elapsed after abiogenesis occurred such that creatures could evolve who are capable of contemplating the frequency of inhabited planets. We don’t know what the minimum evolutionary time scale is (in our case, it was about 3.8 billion years), but if, for instance, this minimum time scale is 3.5 billion years, then it would be impossible for us to find ourselves on a planet with late abiogenesis no matter how rare the abiogenesis process is.
Thankfully, we will soon be able to empirically test the hypothesis that our galaxy is teeming with life. Even a single example of life that had a different abiogenesis event would count much more strongly toward the conclusion that life is common, given the right conditions. Possible examples of life with an independent origin include:
- so-called “shadow life” here on Earth (i.e., life that arose entirely separately from the tree of life, springing from a single root that is believed to encompass all currently known species);
- life elsewhere in our solar system (e.g., on Mars or Jupiter’s moon Europa), if we were confident that there was no panspermia in either direction (i.e., that we didn’t seed, for example, the Martian life via asteroids transporting material from Earth to Mars, nor they us);
- or life on an exoplanet.
Within the next several decades, with rapid progress in biology, with new space missions, and with large and sensitive new telescopes that are planned, it is conceivable that we might find any of these three kinds of inde- pendent life. In this way, we will be able to make a much more informed estimate of the frequency of life in the universe.