Measuring the Cosmos, Mapping the Galaxy, Finding Planets
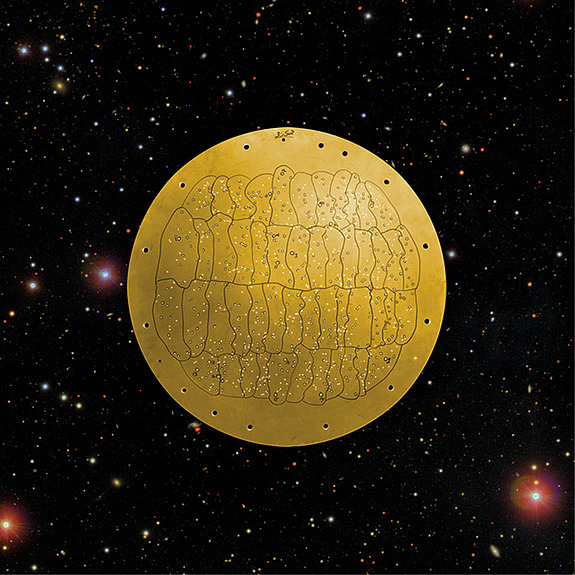
Why is the expansion of the universe speeding up, instead of being slowed by the gravitational attraction of galaxies and dark matter? What is the history of the Milky Way galaxy and of the chemical elements in its stars? Why are the planetary systems discovered around other stars so different from our own solar system? These questions are the themes of SDSS-III, a six-year program of four giant astronomical surveys, and the focal point of my research at the Institute during the last year.
In fact, the Sloan Digital Sky Survey (SDSS) has been a running theme through all four of my stays at the Institute, which now span nearly two decades. As a long-term postdoctoral Member in the early 1990s, I joined in the effort to design the survey strategy and software system for the SDSS, a project that was then still in the early stages of fundraising, collaboration building, and hardware development. When I returned as a sabbatical visitor in 2001–02, SDSS observations were—finally—well underway. My concentration during that year was developing theoretical modeling and statistical analysis techniques, which we later applied to SDSS maps of cosmic structure to infer the clustering of invisible dark matter from the observable clustering of galaxies. By the time I returned for a one-term visit in 2006, the project had entered a new phase known as SDSS-II, and I had become the spokesperson of a collaboration that encompassed more than three hundred scientists at twenty-five institutions around the globe. With SDSS-II scheduled to complete its observations in mid-2008, I joined a seven-person committee that spent countless hours on the telephone that fall, sorting through many ideas suggested by the collaboration and putting together the program that became SDSS-III.
The SDSS uses a dedicated telescope (located in New Mexico) with a 2.5-meter-diameter mirror, similar in size to the Hubble Space Telescope’s, but much smaller than those of the largest ground-based telescopes (whose mirrors are eight to ten meters across). What makes the SDSS special are the exceptionally powerful instruments on the back of the telescope. The first is a giant digital camera—the largest in the world at the time it was built—which has taken deep, multicolor images that cover more than half the northern-hemisphere sky, detecting over 100 million galaxies and 200 million stars. But to measure the distance to a galaxy or the velocity and chemical composition of a star, one has to disperse its light through a prism and identify the fine features etched on its spectrum by individual species of atoms, a kind of observation that astronomers have traditionally done one object at a time. The SDSS took this three-dimensional mapping into mass production by feeding its spectrographs with 640 optical fibers, plugged into 640 precision-drilled holes on a thirty-inch aluminum plate, each hole admitting the light from a single preselected galaxy, star, or quasar. After eight years of operations and more than 2,600 plates, SDSS I and II had measured spectra of nearly one million galaxies, more than one hundred thousand quasars, and half a million stars.
The largest of the SDSS-III surveys (known as BOSS, the Baryon Oscillation Spectroscopic Survey) is aimed at the biggest mystery of contemporary cosmology: the accelerating expansion of the universe. While cosmic expansion was discovered eighty years ago by Edwin Hubble, it had generally been assumed that the expansion would slow down over time because of the gravitational attraction of matter in the universe. In the late 1990s, however, astronomers studying distant supernova explosions found that the expansion of the universe has been speeding up for the last five billion years. Either the universe is pervaded by an exotic form of energy that exerts repulsive gravity—perhaps the “vacuum energy” produced by quantum mechanical fluctuations in otherwise empty space—or else our prevailing theory of gravity itself breaks down on cosmological scales, maybe because gravity “leaks” into extra spatial dimensions that are hidden from our everyday experience.
BOSS will test the “vacuum energy” hypothesis with unprecedented precision, using a novel method that relies on a subtle feature in the clustering of galaxies and intergalactic matter. This feature, the imprint of “baryon acoustic oscillations” in the early universe, has a known physical scale, and after measuring its apparent size (e.g., as an angle on the sky) one can use simple trigonometry to infer the distances to objects that are billions of light years away. Precise determinations—accurate to 1 percent or better—require measuring cosmic structure over enormous volumes, which BOSS will do by mapping the spatial distribution of 1.5 million luminous galaxies and of absorbing gas along the lines of sight to 150,000 distant quasars. BOSS observes fainter objects than the original SDSS, so it required major upgrades to the spectrographs—more sensitive detectors, more efficient optical elements, 1,000 fibers instead of 640—which were installed and commissioned in fall 2009. The survey is now running full tilt and producing its first scientific results. However, the system is very complex, so a typical week still brings a software glitch or hardware problem that generates a cascade of email traffic and telecon discussion, and in rare cases an emergency trip to New Mexico by one of the instrument experts.
Closer to home, two SDSS-III surveys will map the structure and formation history of our own galaxy, the Milky Way. SEGUE-2 (whose acronymic history is too complicated to recount here) focuses on the outer galaxy, which observations and theory suggest was built largely via acts of galactic cannibalism, with the gravity of the Milky Way stretching and eventually destroying infalling satellite galaxies. The SEGUE maps (from SDSS-II and SDSS-III combined) contain about 350,000 stars, revealing partly digested strands of these galactic progenitors. The stellar motions measured by SEGUE also probe the mass and shape of the dark matter “halo” whose gravity holds the Milky Way together.
The inner galaxy is hidden from our view by interstellar dust, tiny smokelike particles that float between the stars and block visible light. APOGEE (the Apache Point Observatory Galactic Evolution Experiment) will map the inner galaxy using an innovative spectrograph that measures infrared light, which passes through interstellar dust nearly unscathed. With the exception of hydrogen, helium, and lithium, all atoms in the universe were forged in stars, then dispersed to the surrounding gas when the stars died. APOGEE spectra will allow separate measurements of a dozen chemical elements—carbon, oxygen, silicon, sulfur, iron, titanium, etc.—for each of the 100,000 stars that it observes. Because different elements form via different nuclear pathways in different kinds of stars, each of APOGEE’s chemical “fingerprints” will encode information not just about the star being measured but about all of the preceding stars that contributed to its composition.
One of the biggest developments in astronomy over the last fifteen years has been the discovery of planets outside the solar system, most of them found via the slight wobble they induce as they orbit their parent stars. Many of the planetary systems discovered to date are very different from our own, with massive, Jupiter-like planets that loop around their parent stars in months or even days, often following elongated elliptical paths rather than the nearly circular orbits that prevail in the solar system. These oddities suggest that many planets “migrate” after birth or undergo chaotic gravitational battles with their siblings. The Sloan survey will, in characteristic fashion, attack this problem with large numbers, monitoring a total of 10,000 stars using a novel, fiber-fed instrument that can measure tiny motions (as small as a few meters per second) of sixty stars at a time. MARVELS (the Multi-object APO Radial Velocity Large-area Survey) hopes to detect between one and two hundred Jupiter-like planets in close orbits, allowing quantitative statistical tests of theories of planet formation and discovering rare systems that may reveal crucial short-lived phases in planetary evolution.
The Institute for Advanced Study helped start the SDSS with a critically timed financial contribution, but over the lifetime of the project its most important contributions have been human ones. Many Institute Members have done spectacular science with SDSS data over the years, and today four of the dozen scientists on the top-level SDSS-III management committee are former IAS postdocs. This is a remarkable statistic for a small institution focused largely on theoretical research. It speaks to the close interaction between theorists and observers in contemporary astronomy—with many individuals who straddle what was once a clear line of demarcation—and equally to the success of the Institute in inspiring its Members to pursue ambitious lines of research whose payoff may lie many years in the future.